Many technologies, such as batteries, fuel cells, ultracapacitors, etc., have been developed for implementation in electric vehicles (EVs) and hybrid vehicles. Among these technologies, lithium-ion batteries have evolved to be the most sought-after ones despite exhibiting some challenges related to safety, cost, and limited energy density. Before diving into buying their first electric mobility adventure, EV adopters share a common primary concern, the vehicle driving range. They are worried that they won’t be able to “refill” when necessary, as they are able to do with gas refuelling, leading to potentially been stranded out of fuel on the road. Unlike gasoline cars, where roadside assistance can be provided with a small amount of fuel, EVs need to be towed to the nearest charging station, costing two things that are often considered premium: money and time. This anxiety is today preventing EV adopters from going all out and their fear is understandable as with the current energy densities of 200 – 350 Wh/kg, only about 300 to 400 kms can be covered per charge. This article addresses what we can do to extend the range through novel battery chemistries.
Nissan LEAF, one of the first EVs in the market, delivered a range of 175 kms per charge and their latest EV has a range of 460 kms. Many vehicles in the market with a range of 300 to 500 kms are available, for example, Hyundai Kona, Volkswagen ID.3 Tour, and Kia EV6. Tesla Model S delivers about 600 km. All these indicate the median range of EVs is steadily on the surge, and all these are possible as the batteries become efficient and affordable. How much distance an EV can drive on a ‘single tank’ depends not just on battery size but also on vehicle speed, how aggressive the acceleration is, the temperature outside, and the usage of AC or heater. Therefore, continuous enhancement in the battery capacity remains a topic of intense research. Battery being an expensive component of the EV, its price decides the market penetration of EVs. To reach price parity with the IC engine vehicles total cost of ownership (includes capital cost, in-operation cost & maintenance cost), that is estimated around $61000 for a 15 year holding period, the cost per kWh should become < $90, which is expected to happen before 2031.
Having said that, based on the statics of average kilometres driven per day, the existing models are more than sufficient for most of our daily commutes, and it is an issue only for intercity travellers and taxis and cabs. To alleviate the fear of EV adopters, “driving to empty (DTE)” is displayed on the dashboard, which is nothing but a moving extrapolated average on the remaining range based on the leftover charge on the car’s battery. DTE is updated continuously based on driving conditions; therefore, it can be considered an indicative value, not a gospel. A battery chemistry which can provide higher capacity, and therefore, range, is Lithium-Sulphur (Li-S) chemistry and S being abundant and low cost, it can potentially reduce the battery cost further.
Working Principle of Li-S battery:
The chemistry of the Li-S battery is quite different from the Li-ion battery, where intercalation of current-carrying Li+ ion into the crystallographic voids of the cathode (generally a transition metal oxide) occurs during charging. As the crystallographic sites are limited, the capacity of the battery is limited by the crystal structure of the cathode. However, lithium reacts with sulphur in Li-S batteries, reversibly forming polysulphide intermediates through a conversion reaction. Therefore nearly 1600 mAh/g of S (practical capacities > 1000 mAh/g) is realizable, which is almost 10 times higher than intercalation-based cathodes. The battery consists of a sulphur cathode and Li anode, with an ether-based organic liquid electrolyte as the ionic conductor. It operates by the successive reduction of sulphur to various soluble polysulphides (Li2Sx, 4≤ x≤ 8) and insoluble lithium sulfides (Li2Sx, 1 ≤ x ≥ 2) at the cathode upon discharge and vice versa during charge (schematically illustrated in the figure below).
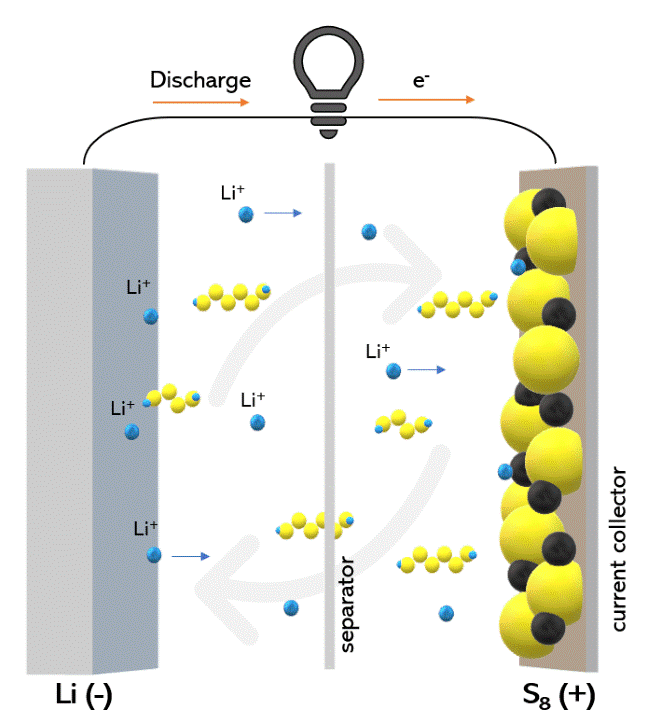
Working principle of Li-S battery
Challenges in the commercialization of the technology
There are several difficulties in the commercialization of the technology; (i) the insulating nature of sulphur (5×10-30 S/cm) which causes very low utilization of active material, (ii) high solubility of S and polysulfide intermediates in organic electrolytes leading to transfer and deposition of sulfide on the anode, and (iii) the volume change of sulphur cathode lowering mechanical integrity of the electrode. Therefore, a solid electrolyte or improved host-guest interaction between S-host and S are actively looked at instead of a liquid electrolyte.
Our group at IIT Madras Research has made many modifications to the cathode materials to trap polysulfides through the physical and chemical affinity. Prof. R. Kothandaraman’s research team at IIT Madrasis working on an effective host material for S anchoring and prevention of polysulfide dissolution. A capacity as high as 1200 mAh/g of S was achieved at 0.2 C rate. A polymer-based solid-state separator is developed to prevent capacity fading, which delivers a stable capacity of 600 mAh/g at 0.2 C for several hundreds of cycles.
Author: Dr. Nandini Jaiswal, Institute Post-Doctoral Fellow, Clean Energy Lab, Prof. Kothandaraman R Group, Department of Chemistry